ALBA Synchrotron
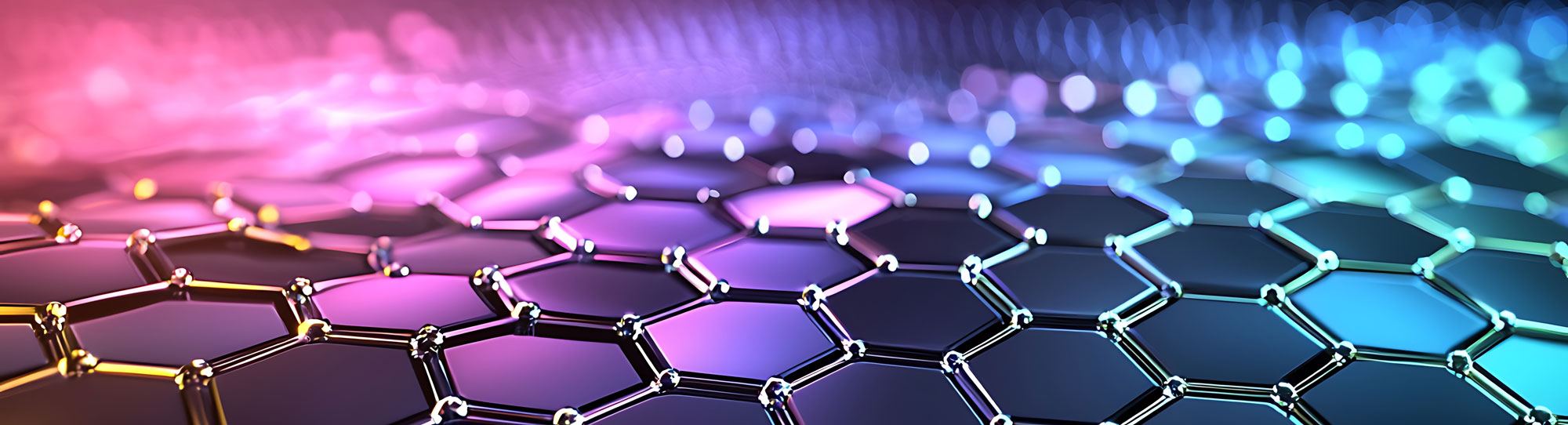
A collaborative study involving researchers from multiple international institutions, including ALBA Synchrotron, has provided key insights into the electronic properties at the contact of two-dimensional (2D) topological insulators and magnetic topological insulators (IMTIs).
The study, published in Materials Today Advances, demonstrates how the epitaxial growth of a bismuth bilayer (Bi-BL) on MnBi₂Te₄-family compounds leads to the formation of hybridized electronic states with potential applications in advanced quantum technologies.
The field of topological insulators has seen rapid advancements due to their unique ability to support dissipationless edge states—electronic channels that can carry a current without energy loss. This property could enable extremely low-power electronic devices for storing and processing information. Magnetic topological insulators, such as MnBi₂Te₄, have garnered particular interest due to their intrinsic magnetic order, which allows for the realization of exotic quantum phases, including the quantum anomalous Hall (QAH) effect and axion insulator states. These materials open pathways for next-generation quantum technologies.
The combination of 2D topological insulators with IMTIs could give rise to new quantum states with tunable Chern numbers, enabling significant control over their electronic properties and greatly enhancing their practical potential. Therefore, this study aimed to synthesize and characterize heterostructures composed of a Bi bilayer on MnBi₂Te₄-family materials.
Through a combination of angle-resolved photoemission spectroscopy (ARPES) and density functional theory (DFT) calculations, researchers found that the bismuth bilayer interacts with the topological surface states of MnBi₂Te₄, leading to the formation of an interface Dirac-like state. While the overall band structure of the bismuth bilayer remained consistent across different IMTI substrates, the mixing of topological surface states was substrate-dependent. The hybridization of Bi and IMTI electronic states suggests that such heterostructures could be engineered to achieve high-Chern-number quantum states and, consequently, with multiple dissipationless edge conduction channels, which could significantly improve the performance of quantum devices.
Synchrotron-based techniques played a pivotal role in this study, particularly at ALBA's LOREA beamline. High-resolution ARPES measurements conducted at ALBA, as well as at Elettra Sincrotrone Trieste, enabled precise mapping of the electronic structure at different photon energies. These measurements confirmed the presence of hybridized interface states and provided critical experimental validation of theoretical predictions for these novel heterostructures. Furthermore, synchrotron light sources facilitated temperature-controlled experiments, ensuring the stability of electronic properties.
This study marks a significant step in the experimental realization of high-Chern-number quantum states through Bi-BL/IMTI heterostructures. The researchers successfully demonstrated epitaxial growth, hybridized electronic states and the emergence of exchange-split 1D edge states, thus laying the groundwork for future explorations into tunable quantum phases.
Experimental core level spectra and fitting data of Bi-5d lines for MnBi2Te4 before (lower spectrum) and after (upper spectrum) Bi-bilayer deposition. Data were taken at a photon energy of 40 eV. Upper and lower insets show corresponding LEED images taken at the primary electron energy of 80 eV. (b, c) ARPES dispersion relations in the wide energy region in the Γ̄M̄ direction of BZ of MnBi2Te4 taken at temperature of 76 K and at a photon energy of 21.2 eV before (b) and after (c) Bi deposition. (d,e) ARPES dispersion relations in the low energy region in the K −̄ Γ−̄ K̄ direction of BZ of MnBi2Te4 taken at 17 K before (photon energy of 28 eV) (d) and after (photon energy of 20 eV) (e) Bi deposition. DFT calculated spectrum (green) is imposed on the left side of panel (e). (f) Constant energy maps in the k∥-space taken at various binding energies. (g) Curvature plot (the procedure is described in Ref. [49]) of the ARPES data at (e).