ALBA Synchrotron
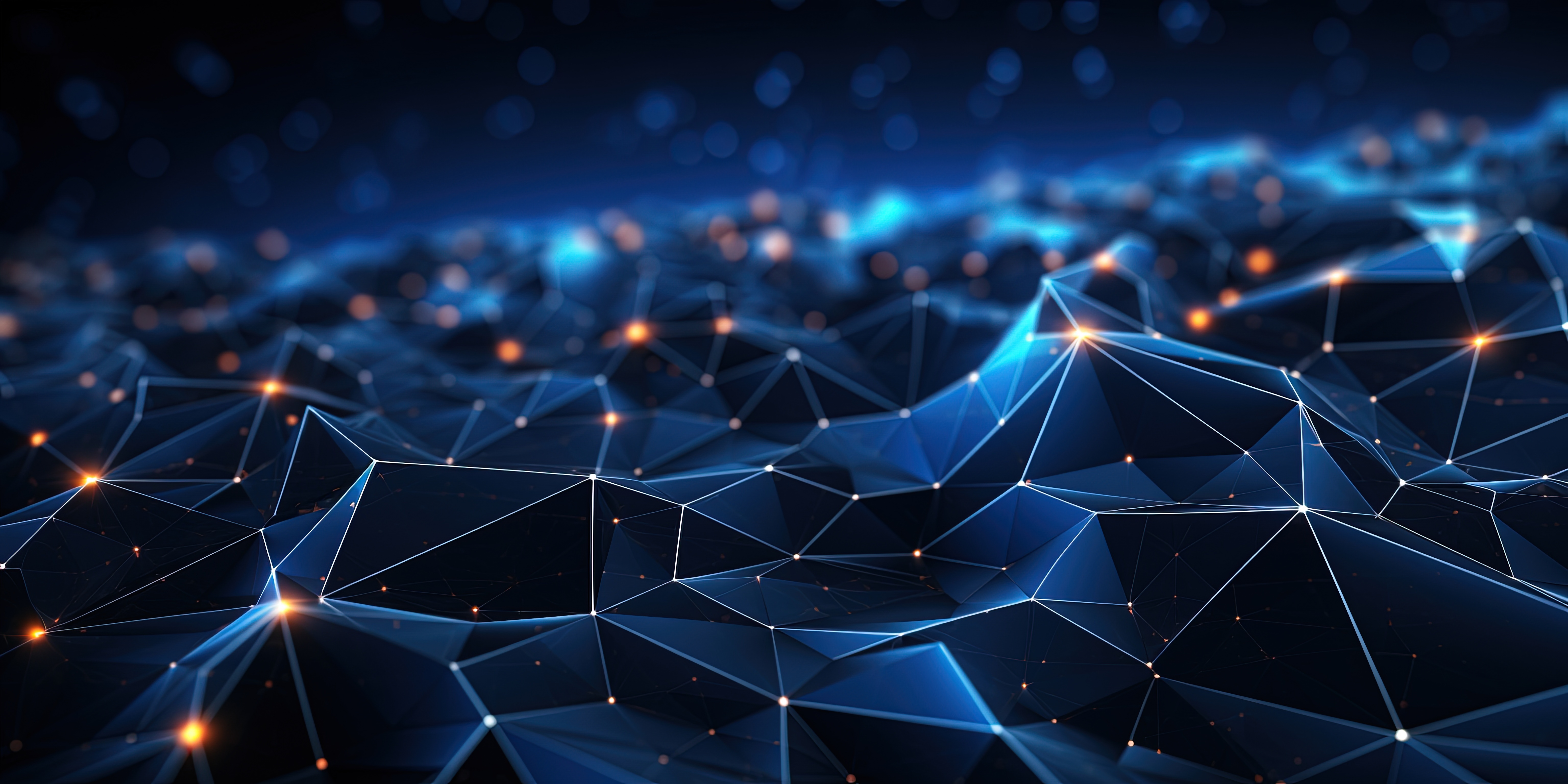
Researchers from the Norwegian University of Science and Technology found a new way to make small, targeted changes in a magnetic system called artificial spin ice (ASI). ASIs are materials with a wide range of emergent properties, such as computation.
Controlling when, where, and how their magnetic domains change can be crucial for technological applications like physical reservoir computing. The new method has been called “astroid clocking” and the main experiment was performed at ALBA, at the PEEM branch of the CIRCE beamline, where the microscope resolves the magnetic state of each individual nanomagnet using synchrotron light.
An ASI (artificial spin ice) is typically an array of small nanomagnets which interact with each other and with external magnetic fields. ASIs are a class of metamaterials (so-called materials of the future), engineered to exhibit unique electromagnetic properties through structured arrangements, differing significantly from the natural behavior of their constituent materials. Recently, ASIs have shown promise for device applications, such as substrates for computation.
These magnetic systems get their name from water ice, where the magnetic moments, or spins, align similar to the hydrogen bonds of ice molecules. ASI nanomagnets are typically blocked (frozen) at room temperature, as the thermal energy is not enough to change their magnetic state. Because there are many magnets, the overall system can have many different states which can be prepared using external magnetic fields.
However until now, these methods have been rather coarse, i.e. they were changing many magnets at a time, in an uncontrolled manner. Or the magnets are written individually, in a non-practical manner, using a scanning probe tip.
Now, scientists from the Norwegian University of Science and Technology have devised a new method called "astroid clocking" that uses a special external field sequence which is able to exactly switch only those elements which are at the border of two regions within the ASI with different states. Thus, it is possible to finely control the state of the ASI array.
The method takes its name from the Stoner–Wohlfarth astroid, a curve that characterizes the critical switching field of a nanomagnet as a function of the angle of the applied magnetic field. By using this information, the method introduced by the research team make use of the magnetic properties of the individual magnets (the Stoner-Wohlfarth astroid) and the interaction between them (dipolar interaction). This approach enables precise targeting of only the border magnets in a given clock cycle. As the border advances in each cycle, the whole array can be addressed.
To perform the study, the researchers from the Norwegian University of Science and Technology prepared the samples to be analyzed at ALBA. The ASIs were iron–nickel alloy (NiFe) on silicon substrate. Once at ALBA, XMCD contrast (X-ray circular magnetic dichroism) analysis were carried out at the PEEM branch of the CIRCE beamline. Its microscope resolves the magnetic state of each individual nanomagnet. Moreover, scientists used was a custom sample holder with a quadrupole electromagnet, a special feature available at this microscope, that allows full control (angle and strength) of the applied magnetic field in the plane. This was the key to realize the field sequence of the astroid clocking. The synchrotron users also developed their own computational code, flatspin, to perform simulations which guided the experiments and helped to understand the results.
This work demonstrates that with astroid clocking, complex spatio-temporal behaviors of magnetic metamaterials become easily controllable with high fidelity. The many states of the ASI system can be considered as units of information for new ways of performing computation, in a different way than today, called reservoir computing. The ASI also provides an interesting model system to study fundamental processes which are otherwise elusive, like magnetic monopoles. With the new way of controlling these systems, both unconventional computation devices and fundamental research benefits.
XMCD-PEEM images of all steps from the relevant unipolar clock protocol series. Time starts at t = 0, and is incremented by 1 for each clock step, with clock pulses indicated by the labels. The black (rightwards) domains grow with application of AB clocking, and quickly reverses with ab clocking. Red circle highlights: the short, vertical domain wall terminating the black domain in the center region of snapshot t = 20 exemplifies both avalanching domain growth and a stuck domain wall. In snapshot t = 21 the top part of the domain wall has progressed in an avalanche to form a finger extension of the domain, while the bottom part of the domain wall remains as before.