ALBA Synchrotron
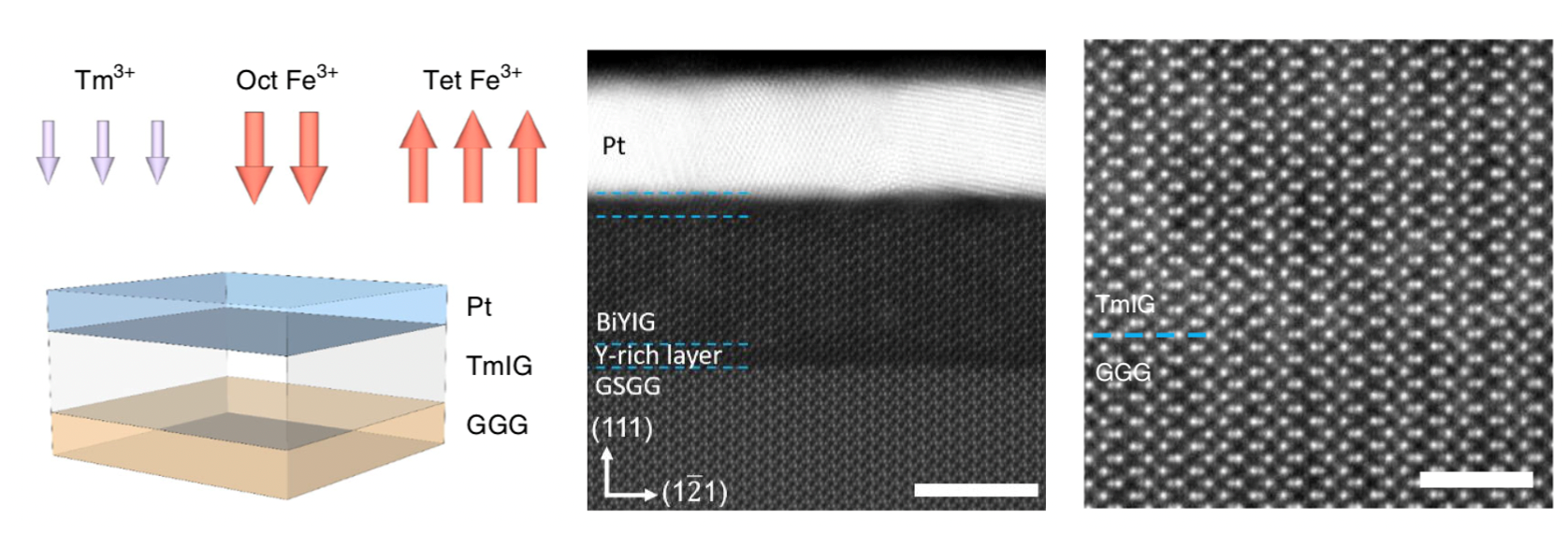
A chiral exchange interaction denominated Dzyaloshinskii-Moriya interaction (DMI) is responsible for exotic chiral and topological magnetic states such as spin spirals and skyrmions. A study lead by researchers at the Department of Materials Science and Engineering at Massachusetts Institute of Technology (US) reveals that in perpendicularly-magnetized iron garnets, rare-earth orbital magnetism gives rise to an intrinsic spin-orbit coupling generating interfacial DMI at mirror symmetry-breaking interfaces. The results have been published in Nature Communications.
Figure 1. Sketch and example of high-resolution TEM imaging of some of the different iron garnet samples of the study, in particular, Tm-substituted iron garnet and Bi-doped YIG structures.
Cerdanyola del Vallès, 24th April 2020.
Inversion symmetry breaking gives rise to rich chiral phenomena that yield new fundamental physics and materials properties. For example, in chemistry, single-handedness of biomolecules results in specialized functionality during biological operations. In particle physics, a fundamental understanding of electroweak interactions hinges on charge-parity symmetry violations. In magnetic materials, a chiral exchange interaction known as the Dzyaloshinskii–Moriya interaction (DMI) can manifest from broken spatial inversion symmetry. This discovery has led to the observation of chiral and topological spin textures, such as magnetic skyrmions and homochiral spin spirals.
In contrast to the well understood case of metallic systems in which spin-orbit coupling arises from a proximate heavy metal, a study lead by researchers at the Department of Materials Science and Engineering at Massachusetts Institute of Technology (US) reveals that in perpendicularly-magnetized rear-earth (RE) based iron garnets (REIGs), rare-earth orbital magnetism gives rise to an intrinsic spin-orbit coupling generating interfacial DMI at mirror symmetry-breaking interfaces.
The study covered the investigation of various rare-earth-substituted iron garnet/metallic heavy-metal bilayers to decouple the contributions of the heavy metal and rare-earth ions to the interfacial DMI (iDMI). The structures were carefully characterized by different magnetic, optical, and transport techniques. In particular, spin Hall measurements to extract the effective fields associated with the DMI and the spin Hall effect present in the samples (fig.2, left).The results demonstrate that iDMI arises intrinsically at mirror symmetry-breaking interfaces due to SOC in the magnetic oxide itself, owing to the orbital magnetism of the RE ion: the iDMI scales with the SOC of the RE ion in the oxide and is undetectable if the RE ion is absent from the iron garnet film. It is also found that the iDMI is influenced by the substrate/oxide and oxide/metal interfaces, but the SOC in the symmetry-breaking metal layer plays little role in the iDMI, and that the iDMI can be tuned by substrate strain by at least a factor of two, providing a powerful means to tune chiral magnetism in oxide materials (see fig.2, right). The XMCD experiments at BOREAS beamline in ALBA were key to directly probe the spin and angular moments, and establish the direct correspondence between the magnitude of spin-orbit coupling interaction and the observed magnitude of iDMI as a function of temperature (fig.3).
The spin-orbit interaction and orbital moment of heavy and rare-earth elements are known to be relevant for spintronic and spin-orbitronic applications.
The discovery
that insulating magnetic oxides incorporating heavy, rare-earth elements, exhibit an intrinsic chiral exchange interaction of Dzyaloshinskii-Moriya type at mirror symmetry-breaking interfaces
opens novel paths towards engineering chiral and topological states in centrosymmetric oxide materials of low magnetic damping, therefore susceptible for very high-frequency and high-speed magnetic applications.
Figure 2: (left) Measuring the DMI effective field 𝑯𝑫 from spin Hall efficiency 𝝌 versus in-plane field 𝑯𝒙. Effective magnetic field from a spin Hall current 𝐻eff acting on a DMI-stabilized Néel DW when a) no in-plane field 𝐻𝑥 is applied, b) small 𝐻𝑥 < 𝐻𝐷, c) 𝐻𝑥 = 𝐻𝐷, d) 𝐻𝑥 > 𝐻𝐷 and e) when 𝐻𝑥 is large enough to flip the chirality of the DW. f) Normalized Spin Hall efficiency 𝜒/𝜒0 as a function of in-plane field, and thus, DW orientation. (right) Summary of DMI (D) strengths in the investigated systems.
Figure 3. (left) X-ray magnetic circular dichroism on GGG/TmIG (6.0 nm). Exemplary Tm XAS spectra at 10 kOe field at T = 70 K for positive (μ+) and negative (μ−) helicities and the XMCD difference spectrum (μ+–μ−); temperature dependence of the XMCD sum-rule derived orbital (Lz) and spin (Sz) moments. (Right) Temperature dependence of the DMI effective field as determined from the spin Hall transport measurements.
Reference: Lucas Caretta, Ethan Rosenberg, Felix Büttner, Takian Fakhrul, Pierluigi Gargiani, Manuel Valvidares, Zhen Chen, Pooja Reddy, David A. Muller, Caroline A. Ross, Geoffrey S. D. Beach. Interfacial Dzyaloshinskii-Moriya Interaction Arising from Rare-Earth Orbital Magnetism in Insulating Magnetic Oxides. Nature Communications 11, 11, 1090(2020). DOI: 10.1038/s41467-020-14924-7