ALBA Synchrotron
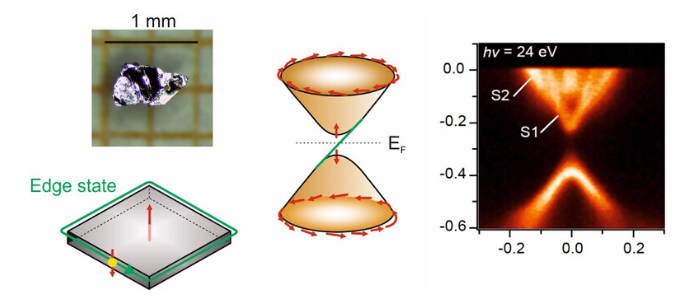
A large group of chemists, theoretical and experimental physicists from Germany, Russia, Spain and Azerbaijan has developed the first intrinsically magnetic topological material. This material is very promising for the development of antiferromagnetic spintronics, 2D magnetism and quantum transport without the need for strong external magnetic fields. Part of the experiments have been performed at the BOREAS beamline of the ALBA Synchrotron.
From left to right, Optical-microscope image of a MnBi2Te4 crystal and a sketch of the expected topological band situation of MnBi2Te4 in momentum space: a single two-dimensional Dirac cone which is gapped due to out-of-plane magnetic order (red) and 1D edge states induced by the non-trivial topology of the magnetic gap (green). Experimental evidence for the presence of a gapped Dirac state on the MnBi2Te4 (0001) surface is confirmed by Angle-Resolved Photoelectron Spectroscopy of the surface electronic structure showing the Dirac state S2 and another surface state S1.
Cerdanyola del Vallès, 19th December 2019.
In quantum materials, a complex interaction between the charge, the spin and the orbital momenta of the electrons add up to exotic macroscopic properties, such as high-temperature superconductivity, giant and colossal magnetoresistance, and quantized conductivity. All too often, very special conditions - like very low temperatures, extreme magnetic fields or high pressure - are needed for these properties to manifest. In a quest to harness the quantum effects for our benefit, researchers are looking for materials that display these properties at room temperature, zero external magnetic fields and atmospheric pressure.
Following the experimental discovery of the first topological insulator (TI) Bi2Te3 in 2009, a conceptually new direction of research on quantum transport has emerged. Nowadays particular efforts are focused on interacting topological phases that exhibit quantum spin transport in the presence of magnetic order or superconductivity. Magnetic topological insulators (MTI) are one particular example. Such systems are envisioned as a hotbed for novel quasiparticles and exotic quantum phenomena, while their experimental realization is very challenging.
Researchers from the Leibniz IFW Dresden and the Technische Universität Dresden have developed the first crystal-growth technique for the first intrinsically magnetic topological material MnBi2Te4. This bulk compound structurally resembles the "patriarch" of all TIs, Bi2Te3, but on top of that it features a periodic sublattice of Mn atoms. These well-ordered magnetic centres establish a 3D long-range antiferromagnetic state below 24 K (-249ºC). As elucidated by theoreticians from the Donostia International Physics Centre (DIPC), Basque Country, Spain, essential ingredients of the topological "flavour" of MnBi2Te4 are its trigonal structure and the interlayer antiferromagnetic coupling. Non-trivial topology manifests itself in the surface states that the experimentalists from the University of Würzburg, Germany, and the Saint-Petersburg State University, Russia, observed independently by advanced angle-resolved photoemission spectroscopy. The experiments at BOREAS using XMCD and XMLD contributed to investigating the magnetic properties and establishing the antiferromagnetic nature of the material.
All pieces of this puzzle are put together in the article published in Nature to confirm that MnBi2Te4 is the first antiferromagnetic topological insulator below its Néel temperature.
The potential implications of this discovery are manifold. Layered MnBi2Te4 heralds a new, post-doping era in the field of MTI, namely, that of intrinsically magnetic stoichiometric compounds and holds promises for antiferromagnetic spintronics, 2D magnetism and quantum transport without the need for strong external magnetic fields (the quantum anomalous Hall effect).
XAS and XMCD measurements showing surface-sensitive magnetic signals under an applied external field and an AFM configuration in the absence of an external field. This data from BOREAS provided critical evidence for the presence of magnetic order at the surface.
Reference: M. M. Otrokov, I. I. Klimovskikh, H. Bentmann, D. Estyunin, A. Zeugner, Z. S. Aliev, S. Gaß, A. U. B. Wolter, A. V. Koroleva, A. M. Shikin, M. Blanco-Rey, M. Hoffmann, I. Rusinov, A. Yu. Vyazovskaya, S. V. Eremeev, Yu. M. Koroteev, V. Kuznetsov, F. Freyse, J. Sanchez-Barriga, I. R. Amiraslanov, M. B. Babanly, N. T. Mamedov, N. A. Abdullayev, V. N. Zverev, A. Alfonsov, V. Kataev, B. Büchner, E. Schwier, S. Kumar, A. Kimura, L. Petaccia, G. Di Santo, R. C. Vidal, S. Schatz, K. Kißner, M. Ünzelmann, C.-H. Min, S. K. Moser, T. R. F. Peixoto, F. Reinert, A. Ernst, P. M. Echenique, A. Isaeva, E. V. Chulkov. Prediction and observation of the first antiferromagnetic topological insulator. Nature (2019). DOI: 10.1038/s41586-019-1840-9.