ALBA Synchrotron
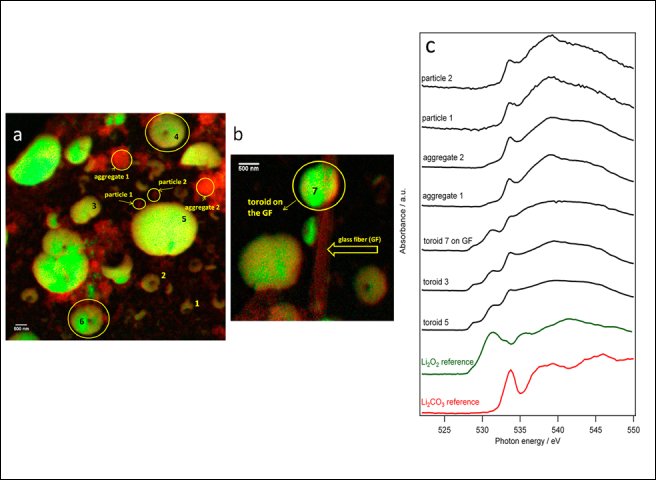
Energy resolved soft X-rays transmission microscopy studies were performed at MISTRAL beamline to know the morphology and composition of the discharge products in lithium-oxygen batteries.
Lithium-oxygen (Li/O2) batteries have a theoretical energy density 5-15 times larger than the well-established Lithium–ion batteries. The management of their apparently simple chemistry is challenging and represents a severe hurdle to the viability of this technology. Fig.1 shows a scheme of the operating principles of the Li/O2 chemistry. In a conventional lithium-ion battery the cathode interface is essentially preserved during operation, in fact the electrochemical reaction is a Li insertion into the active electrode material. Instead, in a Li/O2 cathode the reaction is rather an electrodeposition process, where molecular oxygen from the electrolyte is reduced and combines with lithium cations to form solid products (mainly peroxide Li2O2) that precipitate within the porous electrode:
O2 + 2Li+ + 2e- → Li2O2
When the battery is recharged, the reverse reaction should occur and lithium metal atoms and O2 regenerated. In both reactions the oxygen radicals involved are extremely reactive with the organic environment, and even with the porous electrode (usually carbon), so that parasite reactions will take place. In addition, Li2O2 is an insulator that passivates the electrode surface, and in many cases the reaction cannot be quantitatively reverted because of particles that become electrically disconnected from the electrode. The most relevant issues with lithium−oxygen batteries, i.e. their poor reversibility and cycle life, are mainly coming from these two factors. Therefore information on the complex morphology and composition of the discharge products on the porous electrode are important for the identification of the involved processes.
Fig. 1: Operating principle of a Li/O2 battery (non-aqueous electrolyte). The positive electrode consists of a porous support, usually carbon, which enables the reduction of molecular oxygen and accommodates the insulating discharge products.
Fig. 2: TXM images (a,b) of a carbon coated Au TEM grid after being fully discharged at 100 mA per gram of carbon in a Li−O2 cell. The images are the result of overlapping three color maps with intensities proportional to the amounts of Li superoxide (cyan), Li peroxide (green), and carbonate (red). c) O K-edge XANES spectra at the selected points indicated by arrows in (a,b) and reference spectra measured for Li2O2 and Li2CO3.
To investigate these issues, measurements were performed at MISTRAL which allows to spatially resolve the chemical state of the oxygen atoms thanks to the spectroscopic imaging capability. A common carbon-coated Au TEM grid was used directly as a cathode and fully discharged in a Li/O2battery. On selected areas, 2D absorption images (10 μm × 10 μm) were collected varying the energy across the O K-edge with a spectral sampling of 0.25 eV. In this way, we have obtained 2D maps of the oxygen chemical state of the principal possible reaction products involved, with a full XANES spectrum at each pixel. An example is given in Fig. 2, showing a sample region with Li-O particles of different shape, size and composition. The acquired data have permitted to quantify and localize with spatial resolution of few tens of nanometers the distribution of the oxygen discharge products in these samples (i.e., lithium superoxide, peroxide, and carbonates) and appreciate several compositional, structural, and morphological aspects.
Fig. 3: a) Three-dimensional morphology of a discharged particle showing remarkable deviations from the generally assumed toroidal shape obtained with transmission tomography. b) Sliced view of the same particle shown in a), pointing out that the hole is open only on one side of the particle.
Results confirm the presence of an important amount of carbonates (Li2CO3). On one hand, small irregular particles and aggregates with main carbonate character appeared irregularly distributed on the electrode surface. On the other hand, toroidal shaped particles showed a predominating Li−O phase in the core, which is covered with an external carbonate-rich shell. This indicates that a discharge dominated by objects with smaller surface to volume ratio could benefit rechargeability, and in general suggests that strategies to control the thickness and mechanical and physical properties of this layer should be implemented. The significant LiO2-like phase amounts found in toroids strongly suggest that the formation of these characteristic particles can be related to the availability of large superoxide amounts in the solution phase. Tomographic reconstructions show that in our case deposits have a remarkable distortion from an ideal toroidal shape, rather resembling a mushroom cap, with a central hole open on only one side (Fig. 3).