ALBA Synchrotron
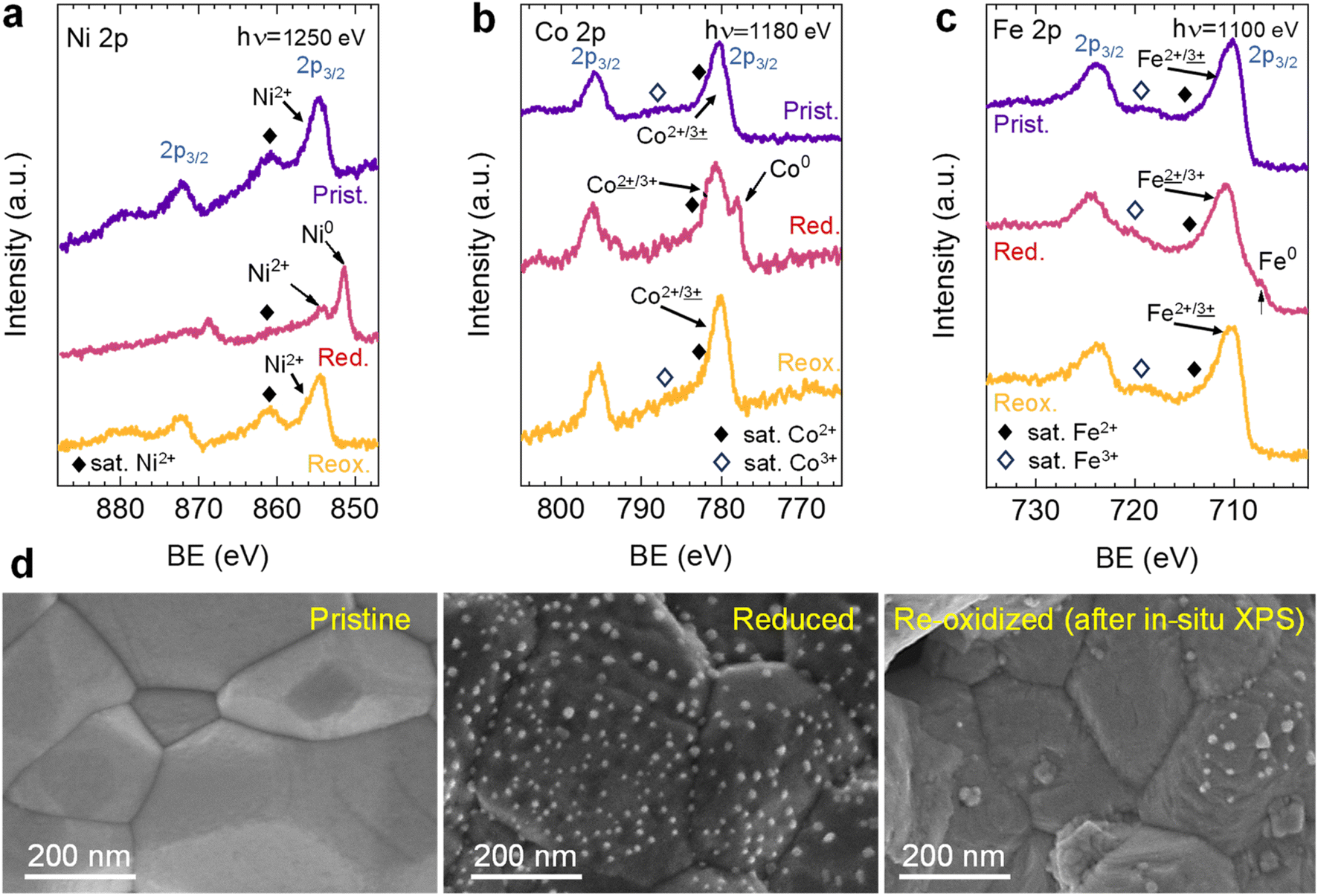
A team of researchers from several institutions in Spain, Germany, and Argentina, led by the ITQ-UPV-CSIC, has conducted a comprehensive exploration of the exsolution process in double perovskite oxides. The study, published in Journal of Materials Chemistry A, reveals important insights into how temperature controls nanoparticle composition, how these particles change during chemical cycling, and provides the first measurement of the reversibility of ternary alloyed nanoparticles.
Advanced X-ray techniques at the ALBA Synchrotron provided detailed views of both structural and surface changes during the exsolution process. This study is of great interest for the development of reversible electrochemical cells that can work in fuel cell and electrolyzer modes for renewable energy storage and production of green fuels.
Perovskite oxides are versatile materials prized for their tunable properties and diverse chemical characteristics, making them exceptional platforms for catalyst design in multiple clean energy technologies, including fuel cells and the conversion of CO₂ and water into CO and hydrogen. Their unique ability to release and—potentially—reabsorb metal nanoparticles makes these materials particularly valuable for creating stable, high-performance catalysts. Exsolution has emerged as a promising nanocatalyst fabrication route in the last decade. Through exsolution, perovskite oxides can produce well-anchored metal nanoparticles under controlled conditions. If this process can also work in reverse, it could enable catalyst regeneration.
However, little is known about how this release-reabsorb process works. For this reason, researchers at Instituto de Tecnología Química (ITQ-UPV-CSIC), the Helmholtz-Zentrum Berlin für Materialien und Energie GmbH (HZB) from Germany, the ALBA Synchrotron, and other research institutions in Spain and Argentina, used advanced X-ray techniques to observe the process in real-time in a quest to understand the dynamics of ternary alloyed nanoparticles. This study specifically examines how three metals—iron, cobalt, and nickel—combine to form nanoparticles within a complex oxide structure, aiming to understand what controls their formation and reversibility.
The researchers investigated the exsolution of ternary alloyed nanoparticles in a specially designed oxide material using a combination of in situ and ex situ techniques. They discovered that the exsolution temperature significantly influences the composition of the resulting nanoparticles. At lower temperatures, nickel-rich nanoparticles preferentially form due to the faster diffusion of nickel. Increasing the temperature promotes the exsolution of cobalt and iron, leading to a more homogeneous composition. This finding highlights the potential for tuning nanoparticle composition by controlling the exsolution temperature.
The study also explored the reversibility of the exsolution process, demonstrating that some nanoparticles can be reintegrated into the perovskite lattice upon oxidation, while others remain on the surface in an altered state. This reversibility has important implications for catalyst regeneration and stability.
Schematic representation and field emission scanning electron microscopy micrographs of the ex situ redox processes affecting exsolution, oxidation and re-exolution.
The research employed two sophisticated X-ray analysis methods using the MSPD beamline and the NAPP endstation of the CIRCE beamline, both at the ALBA Synchrotron. In situ X-ray diffraction (XRD) at MSPD revealed how the material's crystal structure changed during nanoparticle formation. The team observed the material’s framework expanding as oxygen atoms were removed and metals were reduced, followed by the gradual emergence of metal nanoparticles. Initially, nickel-rich nanoparticles formed, followed by the incorporation of cobalt and iron at higher temperatures.
Moreover, in situ near-ambient pressure X-ray photoelectron spectroscopy (NAP-XPS) at the NAPP endstation of the CIRCE beamline provided detailed information about chemical changes at the material’s surface. This technique tracked how different metals changed their chemical state and confirmed the preferential formation of nickel-rich particles at lower temperatures.
Finally, the reversibility of exsolution was investigated by performing reduction-oxidation cycles. NAP-XPS data showed the disappearance of metallic peaks upon re-oxidation, indicating the reintegration of some nanoparticles into the perovskite lattice. However, some nanoparticles remained on the surface in an oxidized and morphologically altered state.
This work advances our understanding of how complex metal nanoparticles form within oxide materials. The ability to control nanoparticle composition through temperature offers new opportunities for catalyst design, and the insights into the reversibility of these processes have important implications for catalyst longevity and regeneration. These findings on how mixed-metal nanoparticles form and transform could enhance clean energy applications, including renewable fuel production at an industrial scale. The research also demonstrates the power of advanced X-ray techniques in understanding complex material transformations, contributing valuable knowledge to the field of catalyst design and development.
Some members of the research team at MSPD beamline, with ALBA director, Caterina Biscari (last right) and the Spanish Minister of Science, Innovation and Universities, Diana Morant (3rd left), who visit the ALBA Synchrotron during their experiment.